On the way to the “quantum steam engine”
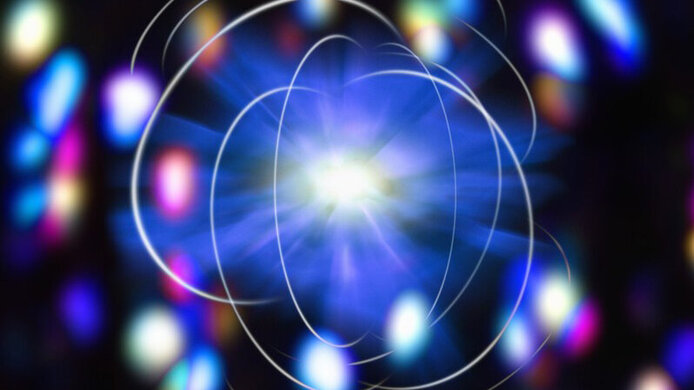
A steam engine uses heat to transform water into its gaseous state. The steam takes up a much larger volume and the pressure that builds up in the sealed vessel can be converted into mechanical work – powering a locomotive, for instance. When steam engines were increasingly used in the 19th century, contemporary researchers started to brood over the underlying general principles. The outcome of these deliberations was the theory of thermodynamics, which can describe relationships between heat, pressure and volume and the associated energy conversion, and provides the basis for many other technical applications.
Thermodynamics is, however, a theory of the macroscopic world. Quantities such as temperature are measured in systems which – like the steam in a boiler – consist of a great number of particles. But what would happen if one tried to apply the questions that thermodynamics answers for macroscopic systems to very small systems? What are the challenges of considering the “thermodynamics of the quantum world”? Would it be possible to build exceptionally powerful quantum machines, in analogy to the steam boiler? These questions are addressed in the international project “Thermal Machines in the Quantum World”, which is supported by the Austrian Science Fund FWF and the German Research Foundation (DFG).
A team of researchers under the lead of Jörg Schmiedmayer and Marcus Huber from the Atomic and Subatomic Physics Institute of TU Wien, in collaboration with partner institutes in Germany, are zeroing in on the research field of quantum thermodynamics, which is only a few years old. “In the microscopic world, there are quantum fluctuations, entangled particles and other effects that seem to contradict the physics of the macroscopic world. When macroscopic processes are studied, on the other hand – when thermal energy is converted into kinetic energy, for instance – one completely ignores what goes on at the level of individual particles,” notes Schmiedmayer. In the context of his project, the team wants to investigate this quantum level in terms of its thermal behavior and prepare the ground for future technical applications. In order to do this, Schmiedmayer and his colleagues will have to devise completely new experiments, and Marcus Huber's group will have to develop new theoretical approaches.
Beyond Ludwig Boltzmann's statistical mechanics
Previously, the connection between the physics of the “big” and the “small” world was described mainly in the theories of statistical mechanics. One of the founders of this approach, Ludwig Boltzmann, was able to relate the processes of the macroscopic world to those of the microscopic world because he described them by way of probabilities. It was not relevant to know the actual location, momentum or quantum mechanical state of individual particles, but rather the probability of encountering these properties.
Huber suspects that if one takes into account actual quantum mechanical processes in systems that produce a power output, the extraction of this power could be much more efficient. There is, for instance, the question of what percentage of the output energy in a machine can be converted into directed energy. “An internal combustion engine has extremely poor efficiency. A great deal of the energy dissipates as heat. If we could introduce a quantum mechanical approach to the way it works, we might be able to change that,” says Schmiedmayer.
Engines, steam boilers, refrigerators and many other machines that are based on the laws of thermodynamics use fuel, steam or coolant as an energy conversion medium. “Again, we may wonder whether this thermodynamic mode of action can be replicated at the level of quantum mechanics. Can a quantum field replace the medium, for instance?” Schmiedmayer continues. “Moreover, in general we have much more information about quantum systems than can be provided by the classical thermodynamic units of measurement. This is why we see great potential in a combination of quantum thermodynamics with theories of quantum information. This approach could result in entirely new ways of looking at things and open up novel possibilities,” says Huber.
Basic research with many open questions
Findings in this research area would have implications for many other research areas. For example, the ever-smaller structures on computer chips must increasingly take quantum mechanical dynamics into account. A theory of quantum thermodynamics could perhaps help with the further miniaturization and cooling of electronic components. And the new fundamental insights could also facilitate the construction of quantum computers.
For the time being, this very young area of basic research is beset by many and possibly groundbreaking questions: can the familiar concepts of thermodynamics such as work or entropy be applied at all in the quantum realm, or must we find new approaches? What does it even mean to measure a temperature locally in a quantum system? How do quantum systems that one brings together exchange thermal energy? “The concepts from classical thermodynamics have only limited validity here,” Schmiedmayer concludes. “We first have to develop new ones for the quantum realm.”
The researchers
Marcus Huber is Professor of Quantum Information and Quantum Thermodynamics and heads the eponymous research group at the Atomic and Subatomic Physics Institute of TU Wien. After positions at the University of Bristol, the University of Barcelona and the University of Geneva, he was made head of a research group at IQOQI, the Institute for Quantum Optics and Quantum Information at the Austrian Academy of Sciences (ÖAW) in Vienna in 2016. In 2015, Huber was awarded a START Prize by the Austrian Science Fund FWF, and in 2021 a Consolidator Grant by the European Research Council (ERC).
Jörg Schmiedmayer is Professor of Atomic and Quantum Physics and Head of the AtomChip Group at the Atomic and Subatomic Physics Institute of the Vienna University of Technology. Earlier career stations took him to Harvard University, the MIT in Cambridge, Mass., the University of Innsbruck and the University of Heidelberg. In 2006, he was awarded the Wittgenstein Prize of the Austrian Science Fund FWF, and in 2012 and 2023 he received an ERC Advanced Grant. Schmiedmayer also represents TU Wien in the Cluster of Excellence “Quantum Science Austria”. A total of five clusters were launched in March 2023 as part of the new Excellence Initiative for Austria. The project “Thermal machines in the quantum world” is set to run from 2022 to 2025 and is awarded EUR 577,000 in funding by the Austrian Science Fund FWF.
Publications
Taranto P., Bakhshinezhad F., Bluhm A., Silva R., Friis N. et al.: Landauer Versus Nernst: What is the True Cost of Cooling a Quantum System?, in: PRX Quantum 4, 2023
Tajik M., Kukuljan I., Sotiriadis S., Rauer B., Schweigler T. et al.: Verification of the area law of mutual information in a quantum field simulator, in: Nature Physics 2023 https://doi.org/10.1038/s41567-023-02027-1
Schwarzhans E., Lock M. P.E., Erker P., Friis N., Huber M.: Autonomous Temporal Probability Concentration: Clockworks and the Second Law of Thermodynamics, in: Physical Review X 11, 011046 – 2021
Gluza M., Sabino J., Ng N. H. Y., Vitagliano G. et al.: Quantum field thermal machines, in: PRXQuantum 030310 – 2021
The project “Thermal machines in the quantum world” is set to run from 2022 to 2025 and is awarded EUR 577,000 in funding by the Austrian Science Fund FWF.