Light source for quantum computers in portable format
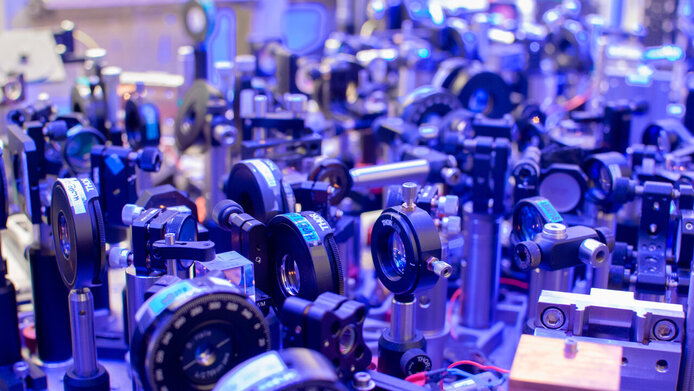
In the context of optical quantum computers light takes on the role that electric current plays in conventional computers: it transports information between circuits. Whereas today's computers only know the states “zero” and “one”, light can also be used to represent a superimposition of the two possibilities. Quantum computers can work with such superimposition states and thus, or so goes the theory, achieve an enormous increase in computing power. Producing and controlling them is still a major challenge, however, as even the smallest disturbance spoils the sensitive quantum effects.
The state of “entanglement” is of particular importance for quantum computers. It refers to objects that are at a great distance from each other, such as light particles, but can still remain connected to each other, an effect that Einstein once contemptuously called “spooky action at a distance”. In the field of quantum optics, where technologies for quantum computers are being developed, this effect, once considered impossible by Einstein, has been used as a matter of course for quite some time in experiments. It has to be noted, though, that the methods used to generate entangled particles used to have a variety of drawbacks, as the physicist Philip Walther from the University of Vienna explains. With the development of a new light source, which he developed as part of a project funded by the Austrian Science Fund FWF, his group has now achieved a breakthrough.
Low reliability
“For the last twenty or thirty years, entanglement has been created by using certain crystals that can manipulate light. A blue light particle can in this way be split into two red ones,” Walther explains. If the procedure is carried out skilfully, it ends with these particles entangled with each other and ready for use in quantum experiments. The downside: “In a crystal, many billions of light particles give rise to only one entangled pair.” According to Walther, this is a problem for quantum computers, which have to process individual light particles and also specifically entangle single photons. This prompted the idea to make the process of creating entanglement more effective.
Atoms provide a solution
The goal can be achieved by having light particles interact with individual atoms instead of crystals and becoming entangled in the process. Under certain conditions, individual light particles can interact with the electrons in the shell of an atom and become entangled with a high degree of reliability. The techniques required to do this are challenging, however, since, atoms are much smaller than the wavelength of light, which results in a very low probability for the light particle to be absorbed by an atom, as Walther emphasises. Several research groups around the world are working on different techniques to achieve higher reliability. “One way is to arrange mirrors around the atom and trap the light particle in them so that it reflects back and forth several times and keeps encountering the atom.” This increases the probability of the light particle being captured. The Viennese physicist Arno Rauschenbeutel, who recently began to conduct research in Berlin, is a specialist in such techniques for coupling light to atoms. Walther realised that Rauschenbeutel's special expertise promised synergies with his own work and could enable him to use atoms to entangle individual light particles.
Light particles in the right colour
To this end, Walther's group had to provide light particles with exactly the right colour, matched precisely to the properties of the atom, so that the atoms would absorb the light. In physics, light is traditionally broken down into its individual colours using a glass prism– comparable to the way white light is split up in a rainbow. A great deal of light is lost in the process, however, as Walther explains, “We deliberately don't try to just filter out the right wavelength as we do with a prism, because that gives you a lot of loss. Our goal was to force a crystal to produce light particles in exactly the right colour.” Walther's experimental setup is similar to that of a laser: light is repeatedly sent through a crystal via mirrors. The crystal generates the desired light particles and reflects them until all the light particles have the right colour.
Portable setup
Walther's group was able to achieve this goal, which they had set themselves before the project began. A light source has now been built that can use atomic systems to generate entanglement. The design of the light source was so successful that various other research groups are interested in cooperating. Walther's group scaled down the experimental setup until it would fit on a plate measuring one meter by eighty centimetres. “We set ourselves the goal of reducing the setup to half the size of a desk, and we managed to do that,” Walther reports proudly. So far, the coronavirus pandemic has delayed the transport of the new source of light particles to other research groups, but as soon as it is possible, the method is designed to be used first by the man who gave them the idea, Arno Rauschenbeutel in Berlin, and later by other groups, such as the Weizmann Institute in Israel.
Patent pending
The success encouraged Walther's team to take a step that is unusual in quantum technology: they filed a patent. “Especially now that more and more companies for quantum computers are emerging, we thought it made sense to protect some elements as intellectual property,” says Walther as an explanation of this move.
Walther's group itself is also working on building quantum computers, albeit of modest size for the time being, and will use the new technology in this context. Developing better quantum computers, however, is not their main goal. “We are not engineers who just want to improve and downsize concepts,” Walther emphasizes. “We like to look at things off the beaten track that no one else has tried yet.” That, as he says, is the task of basic research after all.
Personal details
Philip Walther is a physicist at the University of Vienna and heads the Quantum Computing and Quantum Information Science group. He is interested in quantum technology involving photons, quantum computing, quantum simulation and the interface between quantum physics and gravity. The international project ran for three years, with a one-time extension of six months, and expired in 2020. It was funded by the Austrian Science Fund FWF with EUR 380,000.
Publications
Moqanaki A., Massa F., Walther P.: Novel single-mode narrow-band photon source of high brightness tuned to cesium D2 line, in: APL Photonics, (9), 2019
Massa F., Moqanaki A., del Santo F., Dakic B., Walther P.: Experimental two-way communication with one photon, in: CLEO Pacific Rim Conference 2018, OSA Technical Digest (Optical Society of America, 2018)
Greganti C., Schiansky P., Calafell IA, Procopio LM, Rozema LA, Walther P.: Tuning single-photon sources for telecom multi-photon experiments, in: Optics express, 26(3), 2018
Laudenbach F., Jin R., Greganti C., Hentschel M., Walther P., Hübel H.: Numerical Investigation of Photon-Pair Generation in Periodically Poled M TiO X O 4 (M = K , Rb, Cs; X = P , As), in: Physical Review Applied Vol. 8, 2017