Light pulses at mind-boggling speeds
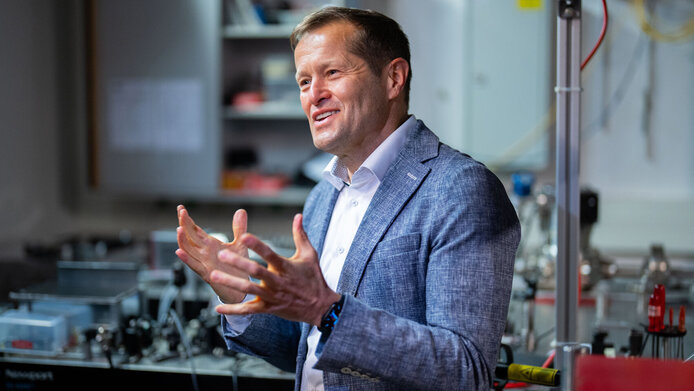
As soon as we hear the pounding of hooves, the horse has already sped past us. The animal is so fast that its hooves are a blur. As we know today, at one point in their movement, galloping horses have all four hooves in the air – they are in full flight, as it were. It was the inventor Eadweard Muybridge (1830–1904) who was able to take the first serial photographs of a horse at full gallop. The Englishman achieved this by stretching wires across a riding track, which were then torn by a passing horse. This activated the innovative electromagnetic shutter release of cameras set up alongside.
With his construction, Muybridge achieved exposure times in the range of a millisecond and opened the door to a new way of exploring nature: slow motion. But even if horses can move very fast, they can't hold a candle to the speeds that prevail in the microcosm.
The clock of an electron ticks in the attosecond range. By using the prefix ‘atto’, experts save some time, as an attosecond is a billionth of a billionth of a second. This period is so short that it was long considered impossible to observe. It took quantum physics, together with modern laser technology, to open a window into the world of attoseconds. Anne L'Huillier, Pierre Agostini, and Ferenc Krausz were jointly awarded the 2023 Nobel Prize in Physics for these achievements. With their ingenious experiments, they are carrying forward Muybridge's legacy.
Krausz, who was born in Hungary, studied physics and electrical engineering in Budapest and at TU Wien (Vienna University of Technology), where he completed his doctorate in laser physics and then qualified as a professor. After winning first the FWF’s START Award and, later, the FWF Wittgenstein Award, Krausz was the first to generate individual attosecond pulses at TU Wien.
Today, Ferenc Krausz is a Director at the Max Planck Institute of Quantum Optics in Garching, Bavaria, and holds the Chair of Experimental Physics-Laser Physics at the Ludwig Maximilian University in Munich. Having remained loyal to the world of ultrashort pulses of light, Krausz is looking at applications beyond the realm of basic research: he wants to revolutionize medicine using attosecond technologies. In our interview, the Nobel laureate talks about his research and the freedom to go where his curiosity leads him.
Ferenc Krausz has developed new methods for generating extremely short light pulses. For his research, Krausz was awarded the 2023 Nobel Prize in Physics. Krausz studied physics and electrical engineering in Budapest and at TU Wien.
Awarded first the FWF’s START Award and, later, the FWF Wittgenstein Award, Krausz was the first to generate individual attosecond pulses in Vienna in order to observe electrons in real time.
Mr. Krausz, your focus is on attosecond physics. What is actually so interesting about the world of the ultrashort?
Ferenc Krausz: In fact, everything in the microcosm is very much accelerated, meaning it is not only very small in spatial dimensions but also in terms of the time dimension. In order to make inroads there, we need the ability to look into such short time intervals. Femtochemistry, for instance, was developed back in the 1980s and 1990s. This field involves observing the formation and breaking up of chemical bonds in real time.
In order for this to happen, entire atoms have to move, which is why this process takes place in the femtosecond range. Electrons, on the other hand, are around a thousand times lighter than atoms, which is why they move at much greater speeds. If we want to capture a ‘still’ of the movement of these particles, our measurement techniques have to be faster, too. In other words, pursuing the goal of generating and measuring attosecond pulses was not an end in itself, but was motivated by a very good reason: enabling us to advance into this previously unknown world of electrons.
So one needs extremely short exposure times to be able to track electron movements. How can you generate such attosecond pulses?
Krausz: In order to generate attosecond pulses, we first need very short, precisely controllable laser pulses in which light ideally passes through only a single cycle. The second ingredient is a so-called non-linearity. Experts speak of non-linear relationships when small changes in one aspect trigger large changes in another. These effects can be observed in special materials, for example, whenever you shine intense laser pulses on them. The light catapults electrons out of atoms or lifts them into the conduction band of a solid – thus making the solid conductive for a brief period.
In both cases, it is possible to limit this excitation of the electrons to the intensity peak of the laser pulse. In this way, you cut out a very small time interval of each peak and trough of a laser wave. If the laser pulse then consists of only a single central wave peak with smaller side troughs, the electrons are excited within attoseconds – and we get an attosecond pulse. Incidentally, this method could also result in a type of transistor that could greatly speed up our electronics by switching electricity with light.
So, you’re saying that in light pulses, the intensity initially increases in every half period of the light wave, is very high for a brief moment and then drops again. If such a half-wave lasts only about one femtosecond, effects that can only occur at the peak of the intensity can have a duration of attoseconds.
Krausz: Yes, and that happens repeatedly, at the peak of all half-periods of the laser pulse. My co-laureates have implemented this approach: using powerful lasers, they were able to first knock electrons from gas atoms within a fraction of the half-wave period, and thus the fraction of a femtosecond, and then hurl them back, creating light waves with multiple frequencies of the original laser. This is a highly non-linear process, but one that creates something like a train of attosecond pulses in extremely short succession. Early in the new millennium, our group succeeded in generating individual attosecond pulses. This is very advantageous for ultra-short time photography in the microcosm, since a camera that has very short exposure times but flashes continuously would come in very handy.
Attoseconds are mind-bogglingly short, much shorter than the time resolution of all known detectors. How could you be certain that you actually generated attosecond pulses?
Krausz: By further developing the principle of the sweeping-image smear camera. The idea behind it has been around for a long time: if a short flash of light falls on a rapidly rotating mirror, the reflected pulse is “smeared” and forms a smear or streak of light on a screen. If the speed of rotation of the mirror is known, the duration of the flash of light can be calculated from the length of the streak. In this way one can achieve resolutions in the microsecond range.
In the next development stage, electrons were detached from a suitable material with the light pulse that was to be measured. The electrons are then deflected by the rapidly varying electric field of a microwave – and thus produce a smear image on a screen. This brings us into the picosecond range, which is still a million times too slow. To finally achieve attosecond resolution, we used light waves to deflect the electrons. In this way, we accelerated the deflection a hundred thousand times again and demonstrated the attosecond duration of the pulses.
How can we learn new things about nature with this technology?
Krausz: Femtochemistry already uses the approach to add energy to a system, such as a molecule, by means of a short light pulse. The pulse causes changes to occur in the system, chemical bonds breaking apart, for example. In order to track such a process, a second pulse is sent shortly after the first one to scan the changes. In this way we can create something like snapshots of extremely fast processes.
They are not the snapshots as we know them from conventional photography, of course. We have not yet reached that level of technology, unfortunately. What we actually do with the scanning pulse is to measure the temporal change of other variables, such as the absorption of the attosecond pulse or the energy distribution of the electrons that the pulse knocks out of the molecule. In the former case, we look at the spectrum of the second light pulse that the molecule has passed through. It will show that certain frequencies are missing that have been swallowed by the system. This gives us information about the current state of the system after it was excited by the first pulse.
By measuring ultrashort light pulses, you have achieved something that no one before has managed to do, and as a result, you have been awarded the world's highest scientific award. What would you like to achieve now?
Krausz: The question of where to go from there actually arose around 20 years ago, after the breakthrough with the first experiments in Vienna. Obviously, you don't just do your work to get a prize. But back then it was clear that we had the tools to look into a world that no one had ever seen in real time.
But before we could use these tools to gain new insights, we had to build confidence in the new techniques. We spent a decade using the new tools to observe processes that were theoretically well understood to ascertain that the new techniques worked well. And they did pass the tests. During this phase, we were also able to improve the measurement technology significantly and we finally knew for sure that it worked. We knew because we experienced processes in real time that were just as the calculations had predicted.
What came next?
Krausz: Our working group then focused intensively on the question of what new findings attosecond measurement technology could produce and how we could use them for something practical. After 2010, we identified two major directions. One was the further development of electronics. We knew that we would have to move from simple atomic systems and molecules to complex semiconductor structures as found in our laptops or cell phones. This means exploring questions of solid-state physics that are as yet unanswered.
One objective is to speed up the microprocessors that are contained in all our gadgets. There has been virtually no progress in terms of clock frequency for around two decades. People managed to achieve further miniaturization, which enabled them to pack a growing number of transistors into the same volume. But in terms of the time dimension, nothing has changed. Our latest experiments on solids have now shown that we can switch electric current on and off even at light frequencies, i.e. a hundred thousand times faster than in today's electronics, by making insulators briefly conductive by means of light.
The second direction involves medical applications. We posed the question of how the interaction between light and matter can be used for medical purposes. Would it be possible, for instance, to extract enough information from human blood to measure a person's individual state of health and to detect diseases – long before symptoms become manifest? In other words, to detect them at a stage where therapies can be applied effectively. That is crucial.
How far away are we from application in this respect?
Krausz: After ten years, we are seeing the first very encouraging results. Using extremely short infrared pulses, we cause the molecules in the blood to vibrate, just like musicians make their tuning fork vibrate. But instead of auditory signals, the molecules emit infrared waves. These are characteristic of the molecules in the blood – they have different oscillation frequencies depending on their atomic composition, and all of this information is contained in this signal. We call this an infrared fingerprint, as the frequencies are captured in the infrared light emitted. They provide a fingerprint-like signature of the individual blood sample of each person.
If these infrared waves can be measured at a sufficient level of sensitivity, the infrared fingerprint will provide a huge amount of information. Ideally, measurements should start when the person is still healthy in order to have a baseline against which deviations caused by emerging diseases can be identified. We have now tested this for the three major disease groups of cancer, cardiovascular and metabolic diseases such as diabetes and have seen that the method works.
When you look back at your beginnings in Vienna in the 1990s: What conditions did you find that made your academic career possible?
Krausz: I owe a great deal to Vienna and the people who were responsible for my career. First and foremost Arnold Schmidt, my mentor and pioneer at TU Wien and later President of the FWF. Essentially, there are three factors that have to be present for successful research: good infrastructure, international exchange, and the greatest possible freedom. Thanks to Arnold Schmidt, we were incredibly well connected at TU Wien. Top researchers, such as the Canadian physicist Paul Corkum, kept coming to Vienna all the time.
These encounters shaped me, and we always knew what was happening at the vanguard of science. Combined with a great deal of freedom, these were ideal conditions. Personal leeway is essential in order to define for yourself which questions you want to answer. That's the most important thing at the beginning, no matter how much time it takes. Once you have found that, it is an inexhaustible source of motivation which carries you through all the ups and downs.
You received the FWF START Award in 1996 and the FWF Wittgenstein Award in 2002. What role do funding agencies like the FWF play on the way to the top?
Krausz: The best idea is useless if you can't translate it into reality, if you can’t afford tools and equipment, for instance. The two FWF grants were enormously important for my research, not only the money in itself, but also the freedom that came with it. In my eyes that doubled the value of the funding. Providing funds with as few restrictions as possible creates ideal conditions for science.
What is your assessment of current cutting-edge research in Europe?
Krausz: One problem I see is the attempt to control research from above along certain program lines. This leads to billions being poured into huge projects, so-called flagships, for research into quantum computing or graphene, for example. It's a bit like a centrally planned economy, subject to political control. I think it would make more sense to use these billions that go to programmatic research to increase the budget of institutions such as the ERC, the European Research Council. I was a member of its decision-making body for many years and my heart bled seeing how many outstanding projects we had to turn down. Not even half of the most outstanding projects could be funded. That doesn't exactly make Europe more competitive in the global competition for the best minds and ideas.
How does excellent science secure our future?
Krausz: US research could not have become so big if it had not been so attractive to the best researchers from all over the world. This is a competition we in Europe must face up to. Only then will unexpected and great things happen. Even if you cannot and should not plan basic research, it is good to define guidelines for yourself. For instance, by reflecting on how to give society the maximum return for the funds it is prepared to spend on research.
We need no new models to do this. The Max Planck Society is a good example. In line with the US model, we are looking for the best minds whom we trust to perhaps one day produce new findings that will benefit humanity. The organization provides as much funding as possible and maximum freedom. The results speak for themselves. In the past five years alone, the Max Planck Society has produced a Nobel Prize laureate each year.
As a Nobel Prize winner, you get a lot of attention. Do you feel you have even more responsibility now?
Krausz: I am indeed getting a lot of inquiries now. From secondary schools, universities, conference organizers. From government circles, too. I am delighted by this interest, which can be used to promote both our field and, perhaps even more importantly, the importance of basic research – far beyond expert circles. In my own working environment, I have always considered it important to pass on my experience to the next generation and to support young colleagues in finding their way. I have been doing that for many years. Since the Nobel Prize, I have had more opportunities to have such discussions also with researchers at the start of their careers.
Thank you for the interview!