Jupiter explorer “Juice” uses Austrian quantum sensor in search for the prerequisites for life
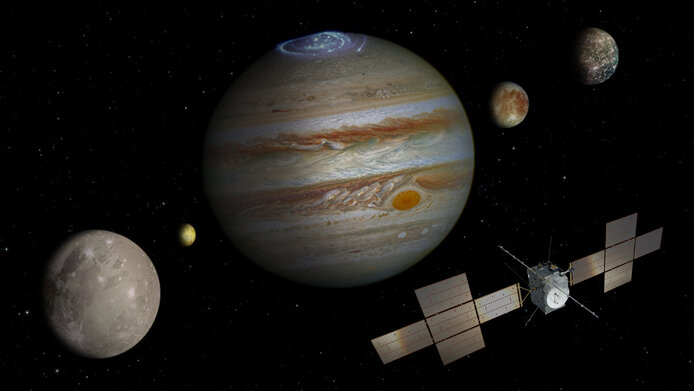
Magnetic fields are everywhere in our solar system. They draw structures into space that are influenced by every medium they pass through – rock, water, plasma, etc. Thus, the magnetic field lines around a planet not only show us what is going on outside a celestial body, but also what is going on inside it: this can relate to its composition as well as to mineral resources or landmines. ESA's “Juice” spacecraft is currently on its way to Jupiter – the largest planet in our solar system. It has a high-precision sensor on board to determine where water, i.e. the prerequisite for life, might be found in Jupiter's moons.
Developed by physicist Roland Lammegger and his team at Graz University of Technology in collaboration with the Space Research Institute (IWF) of the Austrian Academy of Sciences, the sensor named CDSM (Coupled Dark State Magnetometer) uses quantum physics to measure magnetic fields many times more accurately than its classical predecessors. While the previous models also had to be regularly readjusted, the new quantum sensor can determine magnetic fields for weeks on end. Sponsored by the Austrian Science Fund FWF, the “1000 Ideas” project “An optical 3D quantum sensor for magnetic fields” is now intended to enhance the sensor:
“At the moment, this quantum interference magnetometer is a so-called scalar magnetometer. In principle, it 'only' measures the strength of the magnetic field. We now want to transform it into a compass that indicates the field’s direction and strength,” explains Roland Lammegger. Such innovative technology could combine an arsenal of magnetic field sensors in a single device.
ESA's space probe “Juice” took off for Jupiter in April 2023 and is scheduled to arrive in 2031. The quantum sensor developed by physicists at Graz University of Technology will be used to obtain accurate measurements of Jupiter's atmosphere and magnetic field. Researchers are looking for any evidence of potential life on Jupiter’s moons Ganymede, Europa, and Callisto.
Identifying magnetic fields from patterns
The quantum magnetometer on board the “Juice” space probe is based on a gas of rubidium atoms that is irradiated with several coherent light fields. When these fields are precisely adjusted, they collectively put the atoms into so-called dark states, and that changes the transparency of the gas. The frequencies at which less light passes through depend on the strength and angle of the magnetic field. If the frequency of the laser is continuously changed and the absorption is measured, a shadow pattern called the absorption spectrum will emerge. From this spectrum, the researchers can read even the smallest magnetic fields.
The idea behind the sensor can be compared to a partitioned glass plate on which one sets a handful of black marbles. If the plate is tilted, the marbles will be distributed into the partitions depending on the direction and extent of the tilt. By shining a flashlight through the plate from above in the right place, one can deduce the extent of the tilt from the shadow pattern below. Depending on the direction of the tilt, this requires analyzing the pattern at the correct corner of the plate. The process gets much more complex in rubidium gas, of course. In the context of an experiment, the magnetic field is rotated for testing purposes: “When we have reached a certain angle, some absorption lines become smaller, while the others become larger and then we switch electronically to the other absorption lines,” notes Christoph Amtmann, who is involved in the experiment.
The quantum sensor is controlled via five control circuits and must also be installed with a sufficient level of protection for its functions even under the harsh conditions of outer space. The necessary stress tests were carried out at GeoSphere Austria's Conrad Observatory.
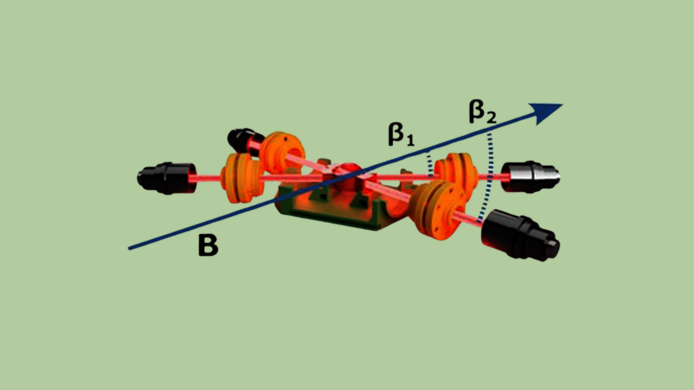
From magnetic field sensor to quantum compass
While the sensor from Austria is on its way to Jupiter – arrival scheduled for 2031 – the group is working on enhancing the measurement technology. At the moment, the scanned frequency range is being adapted to the angle of the magnetic field in order to extract the field strength. In the context of the “1000 Ideas” project, the researchers now want to determine not only the strength, but also the orientation of the field. As this harbors the challenge that some angles produce the same patterns, Lammegger and his team are now analyzing how the geometry of the light fields can be used to expand the sensor: “How many light beams are needed and what must the beams’ orientation to each other be in order to compensate for the angular insensitivities,” wonders Christoph Amtmann.
Moreover, the angle measurements are also more susceptible to external interference factors such as temperature fluctuations. In order to circumvent these factors, the researchers are testing various combinations of settings: “We are changing the sensor temperature, the light output and other parameters of the instrument to find out whether there are parameters where the pattern changes merely by changing the angle of the magnetic field. This is a pretty critical point for us,” notes Amtmann.
A breakthrough could make it possible to replace other systems on board space missions, as the quantum sensor is currently used to calibrate additional measuring devices. Roland Lammegger explains his hopes for future uses of the sensor: “Given that we have the scalar magnetometer on board in any case, we can also use it as an angle-sensitive measuring device. In this way, you only need one magnetometer and it measures angle and scalar values as precisely as an atomic clock.”
The researchers are currently carrying out a wide range of tests to enable this new technology and, thus, produce even clearer images of the interior of our planet in the future.
Personal details
Roland Lammegger heads the “Magnetometry” research department at TU Graz. Christoph Amtmann conducts post-doc research in the same department and at the Austrian Academy of Sciences (OeAW). The researchers are focusing on the development of a magnetic field sensor based on coupled dark states. The 1000 Ideas project “An optical 3D quantum sensor for magnetic fields” (2022-2024) receives EUR 151,436 in funding from the Austrian Science Fund FWF.
Publication
Ellmeier M., Betzler A., Amtmann Ch. et al.: Lower magnetic field measurement limit of the coupled dark state magnetometer, in: Measurement Science and Technology 2024