A handshake between theory and experiment
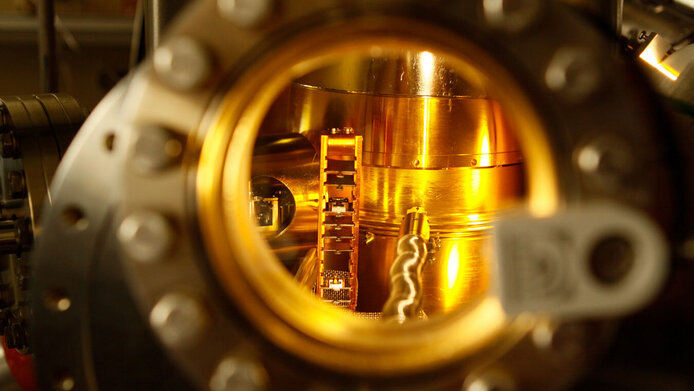
While most metals we encounter in daily life seem to be extremely sturdy materials, many of them are not stable in contact with air – they react with oxygen and form oxides which have extremely diverse properties. Some oxides are chemically very inert, others are the ideal basis for certain chemical reactions, some are perfect insulators, and others again act as superconductors. The field of applications ranges from magnetic field sensors and electronic components to fuel cells. But despite decades of intensive research and pressing technical questions, the exact processes at the interfaces of oxides are still not fully understood. One of the internationally leading researchers in this field, the materials physicist Ulrike Diebold from TU Wien, has now launched a Special Research Programme (SFB) on this topic that is funded by the Austrian Science Fund FWF.
Describing materials atom by atom
Diebold's deputy Georg Kresse notes that it is not the quality of experimental methods that is to blame for the many still unanswered questions about oxides: “We do have experiments where you have very good control and know exactly what is going on atom by atom.” He is talking about scanning tunnelling microscopy, a method that involves revealing individual atoms by scanning a material surface with a fine needle. As Kresse explains, these experiments are important, but the processes that can be observed there often do not correspond to what happens in a material under real-life conditions. Especially when it comes to using the materials in batteries or fuel cells, the concomitant processes are extremely complex. “We ask ourselves: how can we bridge this gap between the exact description of atomic processes and the real-life processes?” The group is using the term handshake-techniques to denote this challenge. To get closer to the real situation, it takes a handshake between well-defined basic-research experiments, experiments in situations close to application contexts, and computer simulations.
Generating smooth oxide layers
The team has recently achieved a first important successful outcome in an experimental activity. As Michael Schmid, a colleague of Kresse’s, explains: “This was about generating extremely smooth layers. Level surfaces are important for some industrial applications, for example in the semiconductor industry or for creating mirrors for high-tech applications. The question was: how do we manage to make layers really smooth at the atomic level?”
Normally, the atoms on oxide surfaces are very stable. “Once they sit at their bonding site, they can only be moved by intense heating, which would destroy the component,” notes Schmid. But in experiments in which the surfaces were exposed to different levels of oxygen concentration, the researchers suddenly observed a change. “At some point, our colleague Giada Franceschi observed that something was going on, and we followed up on it,” Schmid says. The research team found structural changes that depended on how much oxygen was present. “When these oxides go into an oxygen-rich or oxygen-poor phase, the atoms have to rearrange themselves. And when they move, they often do it in such a way as to form a smoother layer in the process.” Hence, the method consists of varying the amount of oxygen offered.
Generally applicable
This effect is special in that it works with completely different oxides. The research group conducted the experiment with iron oxide, oxides of the metals lanthanum, strontium and manganese and indium oxide, all of which have very different properties. Since all the experiments were successful it seems to be a very general effect, and a reversible one at that. While rust, perhaps the best-known metal oxide, is known to be a steadily progressing phenomenon, iron oxide has a very complex structure at the microscopic level and changes continuously depending on whether more or less oxygen is applied. Michael Schmid notes that the process was repeated several times in the experiment in question, “and that's when all the atoms have to start moving. If they are already in motion anyway, they form the type of surface that is energetically most favourable, and that is the smooth surface.”
The two researchers emphasise that this is not only of interest for creating smooth surfaces, but also for avoiding them. Catalysts, for instance, are most efficient when their surface area is as large possible. “You want ramified structures with holes, and you want to prevent what we observed from happening,” says Michael Schmid. This is now facilitated thanks to the new findings.
Machine learning as a game changer
The declared goal of this internationally significant Special Research Programme, which is scheduled to run for several years, is to complement experimental work like this with computer simulations in order to understand the processes in even greater detail. As exact simulations are enormously time-consuming, the researchers based their computer methods on machine learning. This approach brought a breakthrough for their work in the Special Research Programme. “We see an acceleration of some computations by a factor of a thousand to a factor of a hundred thousand, while the quality of the predictions remains the same,” notes Kresse. Although machine learning is currently being hugely hyped at the international level, the researcher emphasises that “the fact that it is being used as a bridge between experiment and theory is new.”
Multiple applications
According to Schmid, there is a wide range of possible applications for the research outcomes. Metal oxides play an important role as catalysts, for example for fuel cells, but also for piezoelectric components. The combination of both methods is also promising. Apart from oxides of iron and exotic metals like lanthanum, the group is also investigating oxides of titanium, manganese, cobalt and strontium. Despite the relevance for high-tech applications, the researchers note that their work has a strong basic-research focus. “The Special Research Programme aims to develop methodologies and establish them internationally,” says Kresse, who does not want this activity to be misunderstood as industry-oriented research. The teams of Kresse and Schmid have more than enough work to keep them busy in basic research.
Personal details
Georg Kresse is the head of sub-project P3 within the Special Research Programme (SFB), which deals with machine learning and quantum simulations, and he acts as co-spokesperson of the SFB. He is interested in the simulation of quantum processes in solid-state physics and is one of the most cited scientists in Austria.
Michael Schmid is deputy head of the P2 department, which reports to the head of the SFB, Ulrike Diebold, and which deals with surface phenomena at the atomic level. He is interested in microscopy methods for studying surfaces, in combination with methods such as spectroscopy.
Focus research at international level
The SFB TACO – Taming Complexity in Materials Modeling is subdivided into nine research sub-projects and receives EUR 1 million per year in funding from the Austrian Science Fund FWF. Scheduled for a duration of eight years, the Programme, which started in 2021, will undergo a progress review after four years, and a decision will then be made on its continuation.
Publications
Franceschi G., Schmid M., Diebold U., Riva M.: Reconstruction changes drive surface diffusion and determine the flatness of oxide surfaces, in: Journal of Vacuum Science & Technology A 40, 2022
Shi J., Li H., Genest A., Zhao W., Qi P., Wang T., Rupprechter G.: High-performance water gas shift induced by asymmetric oxygen vacancies: Gold clusters supported by ceria-praseodymia mixed oxides, in: Applied Catalysis B: Environmental, Vol. 301, 2022
Montes-Campos H., Carrete J., Bichelmaier S., Varela LM, K. H. Madsen G.: A Differentiable Neural-Network Force Field for Ionic Liquids, in: Journal of Chemical Information and Modeling 2022
Reticcioli M., Diebold U., Franchini C.: Modeling polarons in density functional theory: lessons learned from TiO2, in: Journal of Physics: Condensed Matter 2022