7T scanner provides precise 3D maps of brain activity
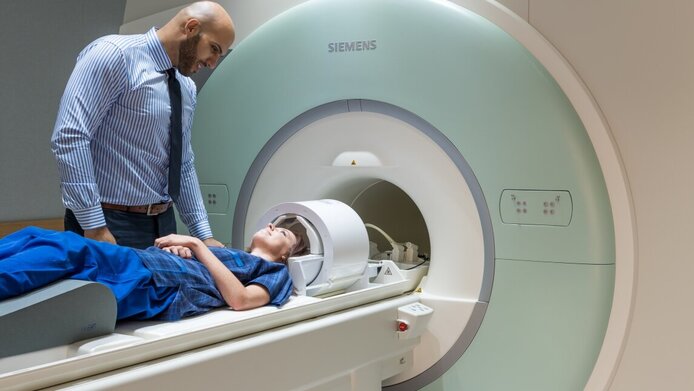
If one is about to cut into a human brain, it is better to have an exact map to hand. The seat of the human mind is richly perfused with blood vessels. Densely packed in furrows and trenches, vital bodily and mental functions are embodied in wired groups of neurons in distinct functional areas. In order to produce a precise map of these areas, the brains of patients are scanned using functional Magnetic Resonance Imaging (fMRI). In simplified terms, images of their brains are recorded at short intervals while they perform various tasks, and the brain activity is mapped in three dimensions. This process is designed to ensure that areas vital for motor function, language and memory remain unharmed when diseased tissue is removed during surgery. The man-machine interaction results in distortions in the images, however, which need to be corrected in order to achieve a perfect match between brain anatomy and brain function.
Highly sensitive in every respect
The MRI scanners currently in clinical use have a magnetic field of 3 Tesla, but the next generation of ultra-high-field scanners with a 7 Tesla (7T) field are already being tested. “7T scanners are capable of an even higher resolution and give more contrast. But they are also more susceptible to distortions which lead to imprecise functional imaging. Hence, we first need to solve the problems of 7T in order to fully reap its benefits”, says principal investigator Simon Robinson, describing the motivation for a research project at the Medical University of Vienna (MUW) that was funded by the Austrian Science Fund FWF. Austria’s only 7T scanner, one of 50 in the entire world, was installed at MUW’s High Field MR Centre in 2008. The higher magnetic field strength provides faster images of the brain functions at a higher resolution. “In this way we are able to see, for instance, whether the language centre has been displaced by a tumour. Unfortunately, the distortions of the magnetic field caused by bones, tissue and air are also stronger with 7T, which has implications for the mapping accuracy”, explains the researcher from the Department for Biomedical Imaging and Image-guided Therapy. Without image correction, the functional areas would not be localised in the brain at the required level of precision.
Individual mapping
The proximity of a number of clinics was essential to realising the project goals, and internal and external project partners contributed expert input relating to physics, programming, clinical fMRI and neurology. For the development of a 7T image correction method, Simon Robinson and his team worked with people suffering from either epilepsy or brain tumours. fMRI depends on the fact that some of the body’s own molecules (haemoglobin in the blood in this particular case) alter the brain’s magnetic field. Countless scans register small changes (e.g. in blood flow or oxygen consumption), thus identifying the areas in the patient’s brain which process cognitive or motor tasks.
New standard for fMRI examinations
During the five year project, the team developed a dynamic image correction process that is an international standard for fMRI studies: for pre-surgery planning, but also for basic research in neuroscience. Before the functional measurements start, the contribution of the scanner to the measured signals is determined precisely. This correction factor is then deducted from the functional images during the image computation. 7T thus helps to create a precise 3D map of individual brains, where functional brain areas are localised exactly within the anatomy of the brain. Neurologists can then decide whether surgery is useful or even possible and which parts of the brain need to be spared at all costs. In a follow-up project, the team intends to develop the method further in order to help determine the best possible location for deep brain stimulation probes in patients suffering from Parkinson’s disease.
Personal details Simon Robinson, Associate Professor at the Medical University of Vienna’s High Field MR Centre of Excellence, focuses on the development of imaging processes for ultra-high-field magnetic resonance (7T). He studied physics at Manchester University, holds a PhD in nuclear physics and has been developing imaging methods for PET and MRI since 1999.
Publications